By Cara Mico
In the second of the climate series I’d like to take a look at ocean conditions.
The Pacific Northwest Aquatic Marine Educators conference recently took place in Netarts, Oregon (more about the conference in follow-up articles). Dr. Burke Hales, Professor CEOAS from Oregon State University, gave the keynote address on the Carbon Cycle in the Coastal Ocean and shellfish production in the Pacific Northwest..
Excess carbon in the atmosphere has many known and well documented impacts. The most commonly understood interaction (at least by the public) is that adding CO2 to the atmosphere increases heat retention. There are so many other interactions from human caused activities and I’m not going to cover them in this article, as entire textbooks dedicated to each barely cover the background.
Instead I’d like to summarize the excellent talk by Dr. Hales on the impact of carbon on near-shore ocean environments.
For millennia, the global carbon budget was in equilibrium. The carbon cycle includes terrestrial, atmospheric, and aquatic habitats. The ocean absorbs roughly 30% of the CO2 released into the atmosphere. Before human industrialization carbon released from plant and animal decomposition as well as from fires would stay locked into the soil or wash away with the rain. The carbon that made it to the ocean was relatively consistent and the organisms in the ocean evolved with this consistent level of carbon.
There was certainly variability and good and bad years for every species. But for the brief period of time that is post-industrial humanity, we’ve tipped this balance in noticeable and measurable ways.
Based on ice core samples, the amount of carbon in the atmosphere is higher than any point in the last million years. There were periods in history that supported life at much higher atmospheric CO2 levels, but it was a planet very different from today’s Earth.
There are issues with using ice core methods to understand ancient atmospheric CO2 levels. First is that the oldest core sample found to-date is only 2.7 million years old, and while researchers speculate that Antarctica may have held ice 10 times older, getting to this ice and interpreting the samples may be impossible with today’s tools. Second, the information we glean from these samples can only go so far. Everything else is pretty blurry.
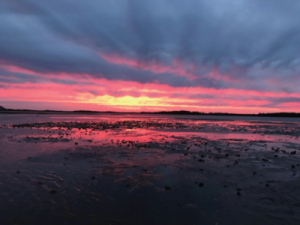
So to understand the impact of rising CO2 levels in today’s ocean we’re left with predictive models, albeit highly sophisticated models. This is where Dr. Hales work fits in.
For several years many shellfish farmers have noticed a reduction in Pacific oyster spawning success, leading to a near collapse of the industry in the Pacific Northwest.
The public latched on to the term ocean acidification after hearing that the excess CO2 in the atmosphere was drastically changing ocean conditions. This is only part of the story.
If you have a basic background in chemistry you’ll know that chemical reactions depend not only on pH, but temperature, salinity, pressure, naturally occurring chemicals, and other factors as well which significantly impacts the rate at which chemical reactions occur.
**** Caution – Gross Oversimplification of Complex Topics Ahead ****
In a strictly controlled environment, CO2 combines with H20 to create carbonic acid or H2CO3. CO2 in solution forms carbonic acid which reacts with carbonate to consume carbonate ions which in turn lowers the pH. This alters the solubility of carbonate minerals, the mineral shellfish shells are made of. Plankton, corals, crab, all rely on calcium carbonate.
Generally speaking, the pH of “pure” water is 7. Rainwater tends to be much more acidic because of the CO2 in the air, with a pH between 5 and 5.5. In areas with heavy industrial pollution sulfur dioxides and nitrogen oxides further reduce the pH to around 4, this is referred to as acid rain. The ocean on the other hand is more basic with an average pH of around 8.1. Keep in mind that the pH scale is logarithmic so a change from 8.2 to 8.1 is a 30% increase in acidity.
But the ocean isn’t a monolith by any stretch of the imagination, in fact categorizing the ocean as a single thing does a disservice to the complexity and diversity of the many bodies of waters that make up the thing we call an ocean.
Oxygen levels, pressure, light, salinity, and temperature can change wildly by moving a few feet in any direction and the stratification provides a variety of habitats for creatures to call home. Think of New York and New Jersey, they seem like they’re so close that they’re basically the same thing but there are people who’ve lived their life entirely in one area and never crossed the bridge to the other. The cultures are completely different.
This article is focusing on shellfish so we’re really talking about nearshore and estuarine habitats.
Back to the research. Dr. Hales and his team wanted to better understand the shellfish collapse in context of pH and marine environment.
**** Findings ****
They found that it’s not just pH that impacts the success of oyster production.
A bit of background about the shellfish in question. Pacific oysters are what is known as broadcast spawners. This means that the male and female reproduction happens in the open water outside the protection of the shell.
By contrast, the native Oregon oyster is a brood spawner meaning that the baby oysters are protected somewhat within the mother’s shell.
Good Pacific oyster spawning conditions arise when the pH, the temperature, the oxygen levels, and the currents are overlapping for the sensitive time period when the Pacific oyster spawn is out of shell in the open water, which is a very short period of time.
When ocean conditions are in thermodynamic equilibrium and oyster spawning is favored, the oysters can survive to adulthood. This state is a constantly shifting balance and incredibly hard to measure. Simplified to the term omega, when omega is greater than 1 the oyster spawn survives to form healthy shells, when omega is less than 1 the brood dies.
The most surprising finding is that while strongly linked to pH, if omega is greater than 1 and pH is acidic, survival rates are still high. So while pH plays a big role, there are other things influencing the life cycle of Pacific oysters.
The models developed by Dr. Hales and his team suggest that pre-industrial conditions had earlier and longer overlaps of ideal conditions, the ideal temperature was earlier and longer and overlapped with omega conditions for greater periods of time. So while there would still have been bad oyster production years, there are more now and the window of opportunity for spawning is shorter.
This only speaks to one species. As you can see we are changing the world around us in complex and difficult to classify ways.
Take the native Oregon oyster for instance, there have been efforts to reintroduce the native oyster to its original habitats to no success, while non-native shellfish proliferate through well-intentioned homeowners seeding docks for future eating, and through accidental introduction from boats and flotsam/jetsam.
If you’d like to hear the full talk we have a video recording available and we’ll post that as soon as it’s processed (keep in mind the speaker’s voice is very low and audience members had trouble hearing what he was saying so be patient). If you’d like to learn more about PACNAME visit their site here, we’ll also provide a bit of background for the rest of the conference as well.
https://www.tillamookcountypioneer.net/climate-change-on-the-oregon-coast-3-part-series/